New smelting processes for titanium
Titanium (Ti) has high specific strength, high corrosion resistance, and abundant resource. However, use of Ti is limited because costs for smelting, melting, and working Ti are higher than that of steel or aluminum. From this background, we investigate new processes for Ti (See below).
1) New smelting process for Ti via. Bi-Ti alloy
Titanium tetrachloride (TiCl4) is reduced into liquid bismuth (Bi) by magnesium (Mg) to form Bi-Ti liquid alloy at 1173 K. In the next step, Ti-rich compound, Bi9Ti8, is recovered by cooling the alloy to 773 K, and pure Ti is separated from Bi by vacuum distillation. In this process, the production speed is expected to be faster than the current commercial process, and Ti powder can be produced.
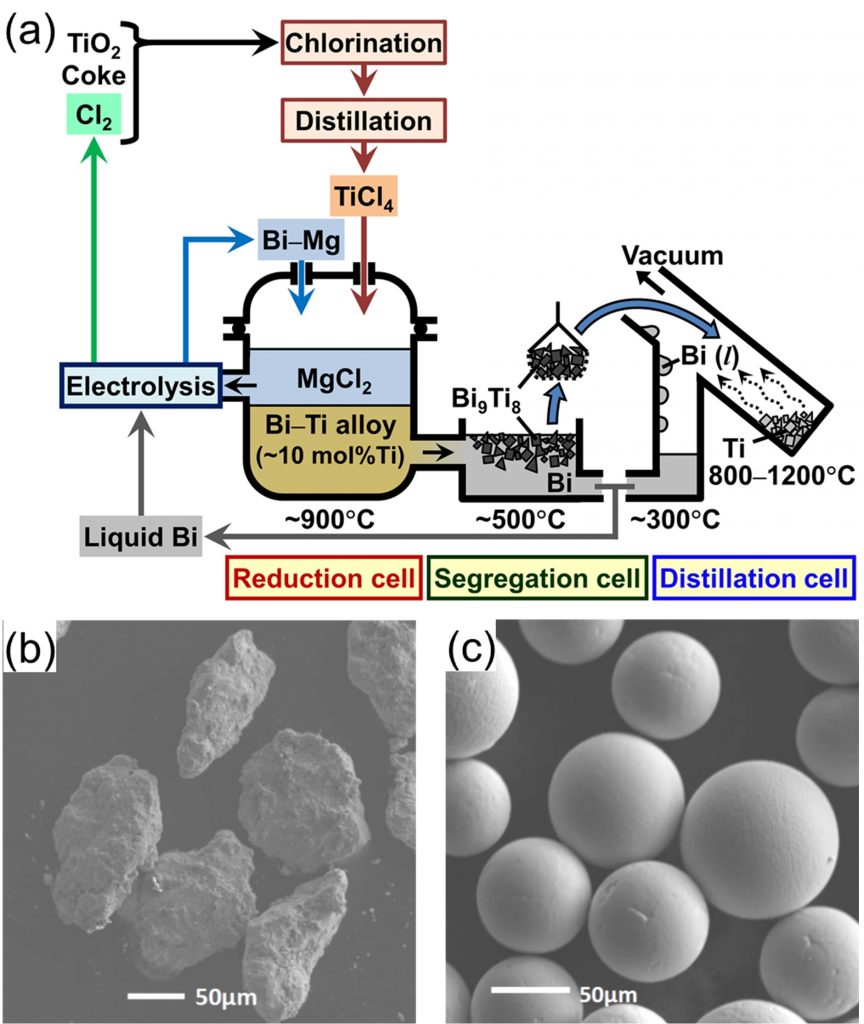
(b) Ti powder obtained by distillation of Bi-Ti alloy and (c) Ti powder after PDR
2) Direct production process for Ti sheet by electroplating in molten salt
Electrodeposition of Ti is carried out in TiCl2-added molten halides, such as magnesium dichloride, sodium chloride, and potassium chloride, at high temperature in the new process. Ti is flatly and smoothly deposited by the electrolysis and peeled off from cathode substrate. Our group demonstrated flat electrodeposition of Ti with simple equipment and peeling off Ti sheet from substrate.
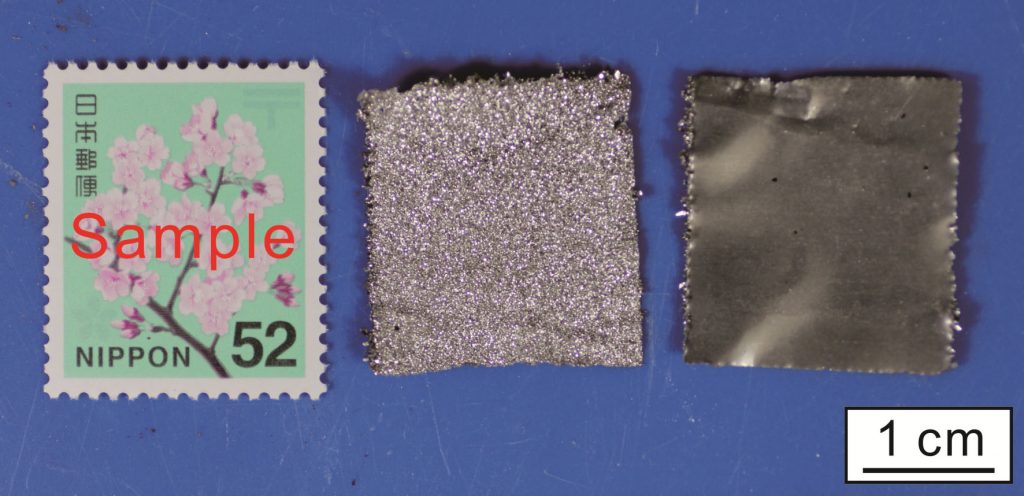
Protonic ceramic fuel cells (PCFCs)
Because of potential application as efficient power generation devices, protonic ceramic fuel cells (PCFCs) operating in the intermediate temperature range (400-600 ℃) are receiving increasing attention. We have developed PCFCs using yttrium-doped barium zirconate (Y-doped BaZrO3, BZY) with both high proton conductivity and excellent chemical stability, which is regarded as the most promising candidate as the electrolytes in PCFCs. In our studies, the ionic conductivity of BZY has been enhanced up to 30 mS/cm at 600 ℃. In addition, we have succeeded in fabricating an anode-supported cell with high performance (open-circuit voltage: 1.03 V, peak power density: 172 mW/cm2).
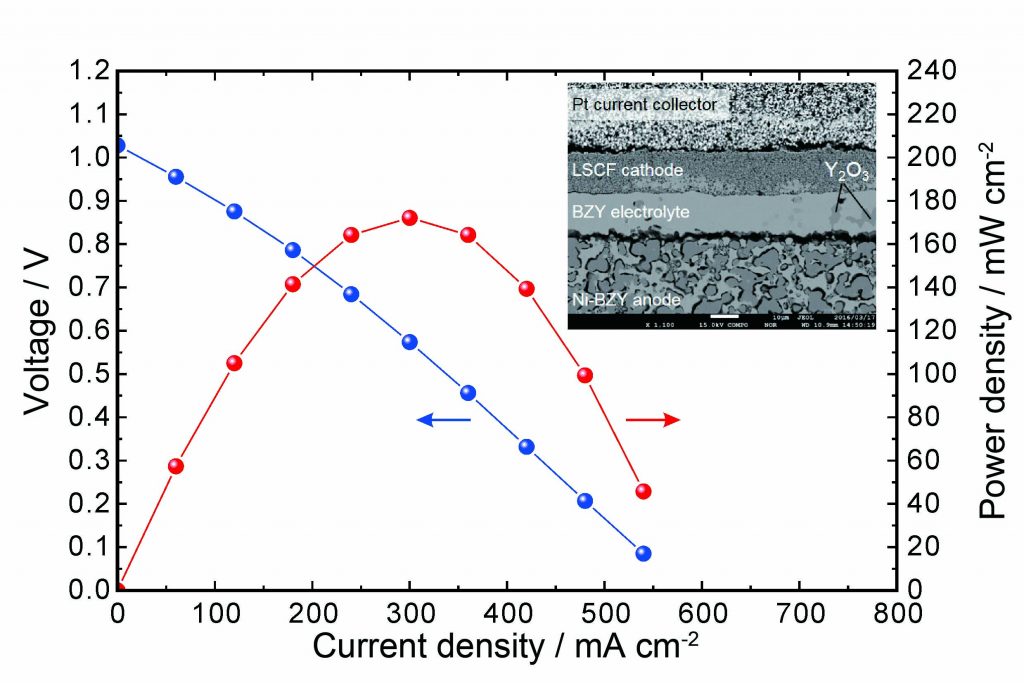
A machine-learning method of efficient PES mapping for analyzing ionic conductivity
The mobility of an ion in a host crystal is fully characterized by the entire potential energy surface (PES) of the mobile ion. Although the entire PES can be theoretically evaluated by exhaustive local structural optimizations around the mobile ion, such an evaluation requires huge computational costs, particularly by first-principles calculations. We have therefore developed a machine-learning (ML) method of efficient PES mapping with several collaborators, in which only the global minimum point and the bottleneck point on the optimal path are evaluated selectively (See below).
The basic strategy for identifying the two dominant points is to construct a probabilistic Gaussian process (GP) model of the entire PES, which is iteratively updated using the first-principles PEs already computed in the earlier steps. The next point for PE computation is selected by the estimated likelihoods of the dominant points within a Bayesian optimization (BO)-like framework. The intrinsic difficulty is that the optimal path and its bottleneck point are found after acquiring complete information about the entire PES. Use of only the mean and variance at each grid point is never sufficient to estimate the likelihood of the bottleneck point, although these are usually used in typical GP + BO strategies. To overcome this difficulty, multiple randomized PES samples were generated according to a probabilistic GP model, and the optimal path for each PES sample was identified using a dynamic programming (DP)-based algorithm. This enables collections of the global minimum and bottleneck points to be obtained, and these collections are considered to be distributions representing the likelihoods of the dominant points.
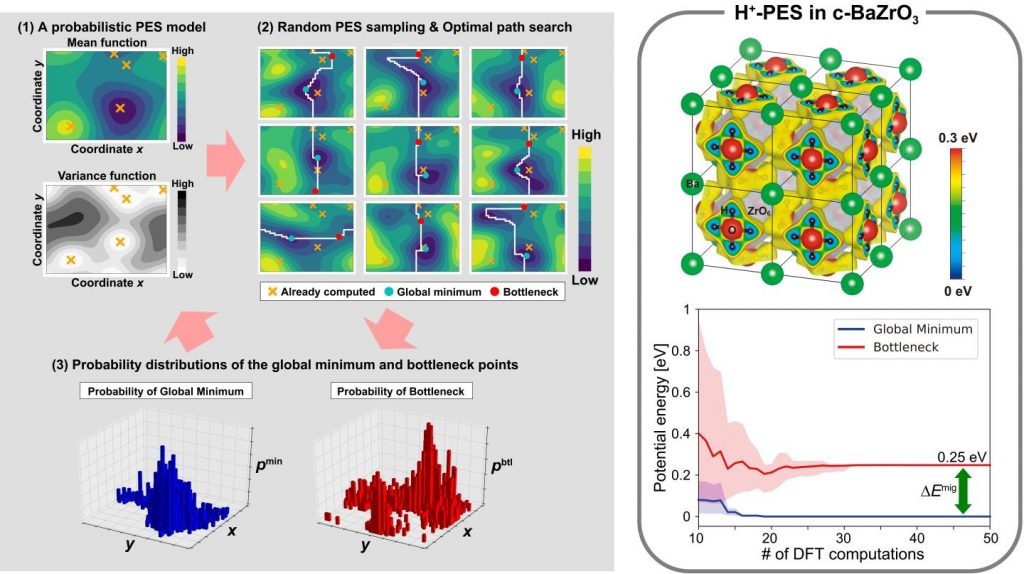
Thermochemical energy storage materials for the effective use of waste heat
Factories and power plants emit large amounts of waste heat at low temperatures below 250°C. The use of fossil fuels can be reduced if waste heat can be stored using thermal energy storage technology and used effectively where and when it is needed. Therefore, we are focusing on thermal energy storage technology that uses heat from chemical reactions. To achieve this, it is necessary to find a chemical reaction with a reaction temperature suitable for the waste heat, a high reaction rate, and sufficient heat storage density. We have found for the first time that the hydration and dehydration reactions of rare-earth sulfates proceed rapidly in the temperature range of 100-250 °C. Experiments and computer simulations have shown that the dehydration and hydration reaction of this material is driven by the insertion/desertion of water molecules into the crystal structure. We are now investigating the mechanism of this reaction in detail, and are aiming to develop new thermochemical energy storage materials based on the knowledge obtained.
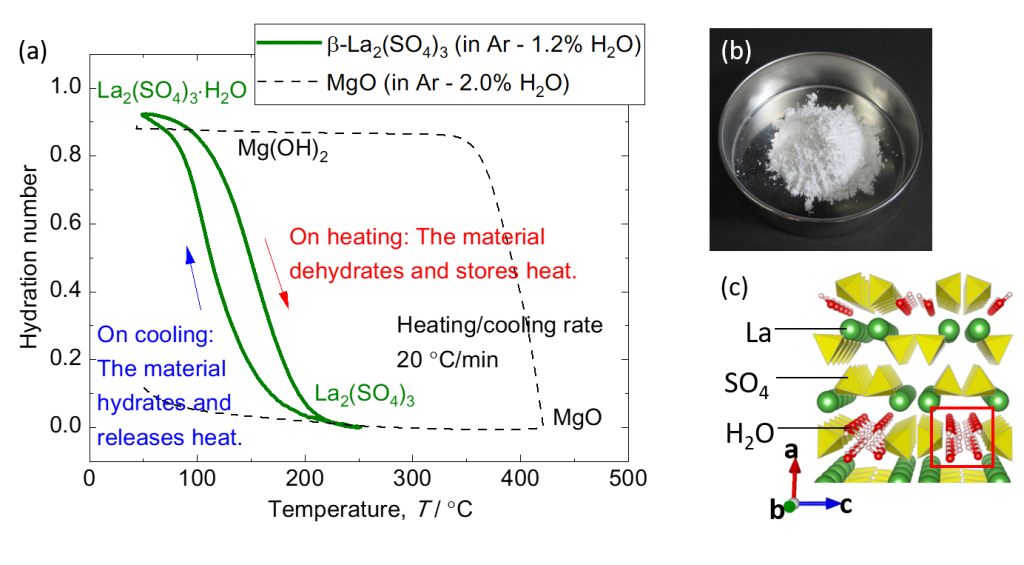
(c) Crystal structure of lanthanum sulfate monohydrate. There are one-dimensional diffusion paths for water molecules.